Tom Royston
Tom Royston
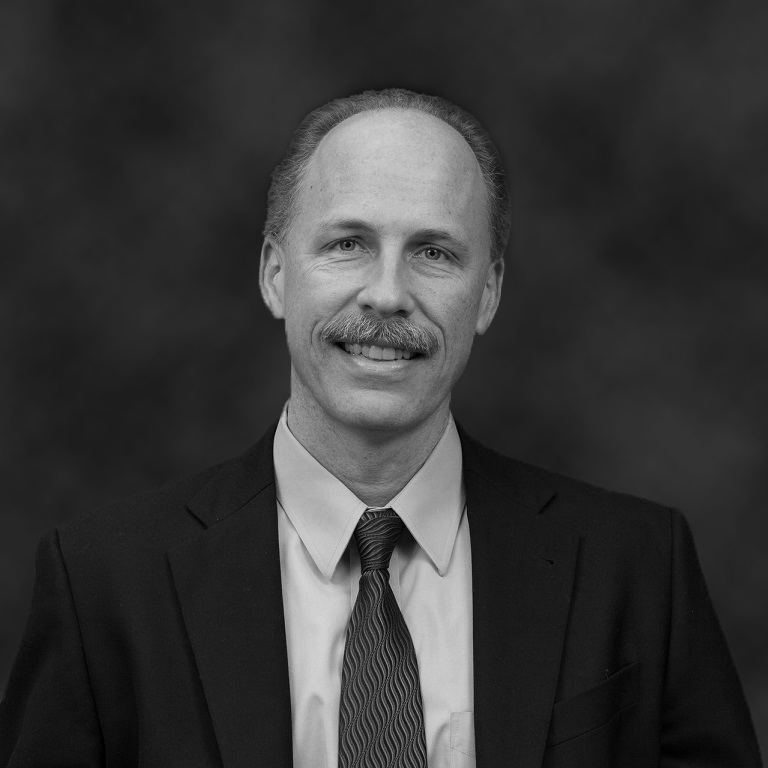
Biomedical Engineering Faculty
Profile
Thomas J. Royston, Ph.D., Professor and Head of Biomedical Engineering at the University of Illinois Chicago (UIC) has been a faculty member at UIC since 1995, with appointments in Biomedical Engineering and Mechanical Engineering, and head of Biomedical Engineering since 2009. Tom’s NIH and NSF-supported research in mechanical wave motion and imaging in porous and nonporous viscoelastic materials, and acoustics applied to medical diagnostics and therapy has been recognized with the NSF Career Award, the NIH NIBIB Nagy Award and the Acoustical Society of America (ASA) Lindsay Award. He is a Fellow of the American Society of Mechanical Engineers (ASME), the American Institute for Medical and Biological Engineering (AIMBE), and the ASA. He has served as associate editor for journals of the ASA and ASME, and is a founding member of the International Society for Magnetic Resonance in Medicine (ISMRM) Magnetic Resonance Elastography Guidelines Committee. He has authored over 100 peer-reviewed journal articles, and 200 additional conference and other publications.
- View full profile: https://bme.uic.edu/profiles/thomas-royston-phd/
Description
#1) Design and Test small animal ventilator system for Hyperpolarized (HP) Xenon MRI
HP 129 Xe(non) MRI is an emerging imaging method primarily for assessing the pulmonary system. UIC is developing preclinical and clinical capability in this novel imaging technique, which requires inhalation of a “pre-treated” xenon gas mixture. UIC is a leader in pulmonary research, from basic science studies utilizing mouse models of a range of pulmonary pathologies through human subject studies as part of translational and clinical trial studies to develop new biomarkers and disease treatment protocols. Human subjects can be instructed to inhale this mixture and hold their breath during the image acquisition. For animal subjects ventilation of the gas needs to be controlled. There are unique challenges to providing controlled ventilation of HP Xe gas to a mouse that require in-house technical development. The Summer BEST participant will be involved in developing and testing a ventilator for this application.
- Visit lab website: https://acoustics.lab.uic.edu/team/